Science with IceCube-Gen2
Neutrino and multimessenger astrophysics
Searches for neutrino sources
In 2018, IceCube announced for the first time that an astronomical source of high-energy neutrinos had been identified after the detection of neutrino emission from blazar TXS 0506+056 in coincidence with gamma-ray radiation from the same source seen by Fermi. In 2022, an analysis of ten years of IceCube data observed neutrino emission from the nearby active galaxy NGC 1068.
These were exciting first results that proved that neutrino astronomy is possible. But there is much more to explore. The new IceCube-Gen2 facility will greatly enhance the pointing and resolution capabilities in the search for sources of high-energy neutrinos.
The IceCube-Gen2 optical high-energy extension will provide more astrophysical neutrinos by a factor of ten, enabling the detection of five times fainter neutrino sources compared to current technology. From supernovae in our galaxy, for which IceCube provides the best statistics for measuring the neutrino light curve, to the broadening of multimessenger detections of the most extreme environments—through joint detections with other facilities using cosmic rays, gamma rays, other high-energy electromagnetic radiation, or gravitational waves—the opportunities for discovery are powered up with IceCube-Gen2.
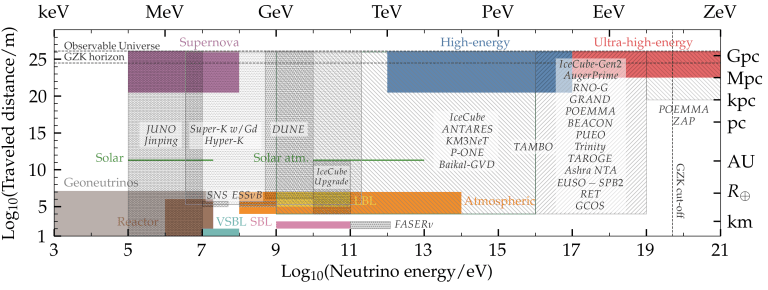
In addition, the planned approximately 500-kilometer radio array will extend the energy range of IceCube-Gen2 to the EeV scale. Based on the current measurement of the diffuse neutrino flux, this detector would allow not only the observation of particles from the huge cascades created by ultra-high energy cosmic rays (UHECRs) interacting in the atmosphere, but also the first detection of GZK neutrinos, also known as cosmogenic neutrinos, which originate directly from the interaction of the highest energy cosmic ray protons with the cosmic microwave background near the source.
Finally, IceCube-Gen2 will expand the detection reach for core-collapse supernovae by using their high-energy neutrino emission, thus further exploring the megaparsec range initially explored by IceCube—an order of magnitude increase in the number of observed neutrinos is expected, which would scale the detection horizon by a factor of 3. IceCube-Gen2 will also be sensitive to the burst of low energy neutrinos expected to be produced during the supernova. Its high uptime and low detector noise make it a valuable asset, providing an alert system for what is expected to be a once-in-a-lifetime event.
High-energy astrophysics
Although the detection of a source sparks the interest of people of all ages, the reality is that a deep knowledge of our universe also draws on other less popular astrophysical measurements.
The improved statistics and larger energy range provided by IceCube-Gen2 will allow better characterization of the high-energy neutrino and cosmic ray spectra as well as the flavor composition of neutrinos over a wide energy range. These are important measurements for understanding cosmic ray acceleration mechanisms and for a global comprehension of how the various cosmic accelerators contribute to the high-energy radiation in our universe.
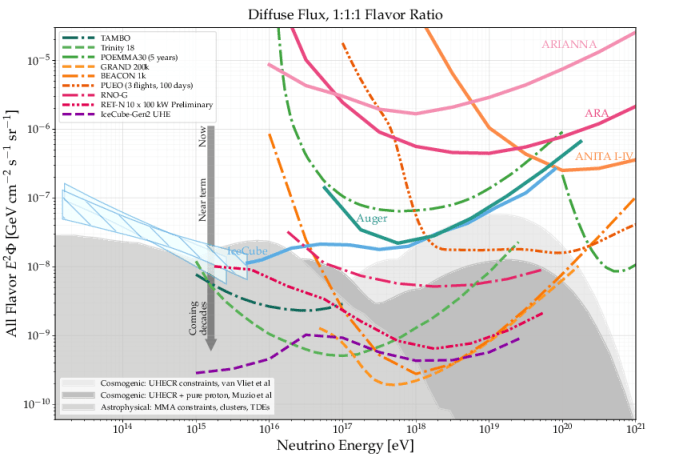
These studies provide unique insight into the dense environments of the most powerful cosmological sources, which cannot be probed directly by other means, since light or other sorts of electromagnetic radiation is trapped by interactions with nearby particles. All this knowledge, in turn, feeds cosmological models and helps to further our understanding of the evolution of the universe.
IceCube-Gen2 will provide accurate and unprecedented measurements of the cosmic-ray anisotropy in the region of 30 PeV to several EeV, allowing the study of the transition from galactic to extragalactic sources.
The existence of dark matter and its impact on the source composition and radiation spectrum of the extreme universe is also an area of research to which IceCube-Gen2 is expected to provide outstanding contributions.
Neutrino physics
Although IceCube was initially conceived as a neutrino telescope to explore the extreme universe, it was soon realized that high-energy neutrinos provided by nature could also be used for particle and fundamental physics research.
With IceCube-Gen2, the future South Pole neutrino observatory—which encompasses both the original IceCube and its low-energy extension, the IceCube Upgrade—will be able to measure neutrinos in the largest energy range provided by any scientific facility, from a few GeV up to the eEV scale.
Thus, the next-generation high-energy extension makes IceCube a unique environment for precision measurements in neutrino physics as well as in the search for new physics or the ultimate probes of fundamental physics.
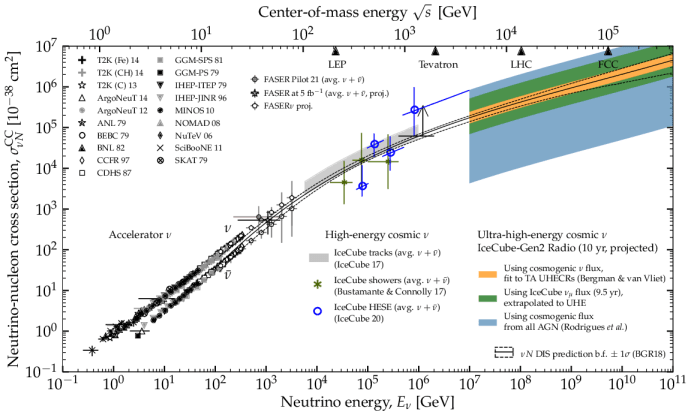
And, finally, the largest fluxes of astrophysical neutrinos, in the TeV-PeV region, will enable more precise measurements of the high-energy neutrino cross section as well as flavor ratio cross sections and will also expand these measurements to the highest energies ever.
It is in this region that IceCube discovered a Glashow resonance in 2021 and that IceCube-Gen2 will enable more precision measurements of W-boson mediated interactions.
The new capabilities of IceCube-Gen2 will facilitate searches for dark matter, extra dimensions, leptoquarks, and other new particles, or even new fundamental symmetries and ways to explore the energy scales where non-perturbative QCD effects are expected to start becoming important.
Earth sciences
The properties of the Antarctic ice itself are an essential study for IceCube-Gen2, as the calibration and thus the pointing and energy resolution of its detectors depend heavily on our deep understanding of the medium in which our sensors are embedded and through which neutrinos and other high-energy particles travel.
In addition, occasions to drill the ice sheet almost to its bottom, which rests on the Antarctic bedrock, are rare and provide unique insights not only into the properties of the ice but also into what the configuration of this ice and the impurities it contains can tell us about the Earth’s past history.
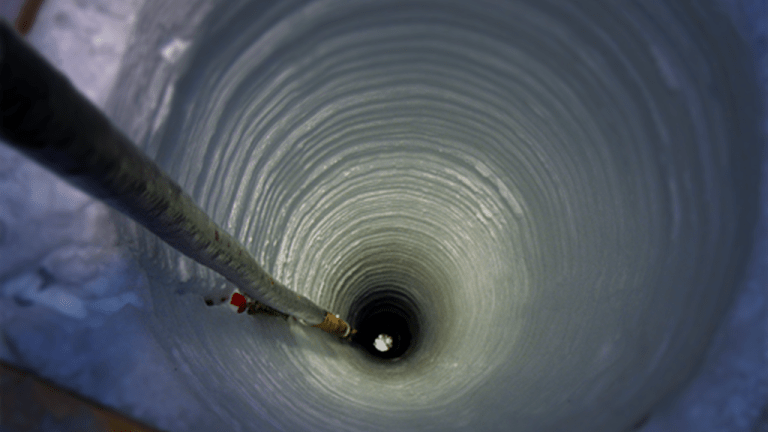
These are the reasons behind dedicated efforts within IceCube-Gen2 devoted to broaden its science scope towards glaciology and other Earth sciences, such as paleoclimatology or even the study of microbial life in these extreme ecosystems.
Outcomes of these efforts are already feeding decisions on the design of the IceCube-Gen2 drill system as well as the processes to deploy the new sensors to minimize the impact of the melting-freezing cycle.
And the collaboration, with experts in the field of glaciology, will continue throughout the deployment years—with light sources and laser probes deployed on board or in parallel to IceCube sensors—exploring the purest and largest ice sheet on Earth.